Following are some of the terms used in topology which have similar definition or literal English meanings:
- Convex set: A subset
of
is called convex1 , if it contains, along with any pair of its points
, also the entire line segement joining the points.
- Star-convex set: A subset
of
is called star-convex if there exists a point
such that for each
, the line segment joining
to
lies in
.
- Simply connected: A topological space
is called simply connected if it is path-connected2 and any loop in
defined by
can be contracted3 to a point.
- Deformation retract: Let
be a subspace of
. We say is a
deformation retracts to
if there exists a retraction4
a retraction such that its composition with the inclusion is homotopic5 to the identity map on
.
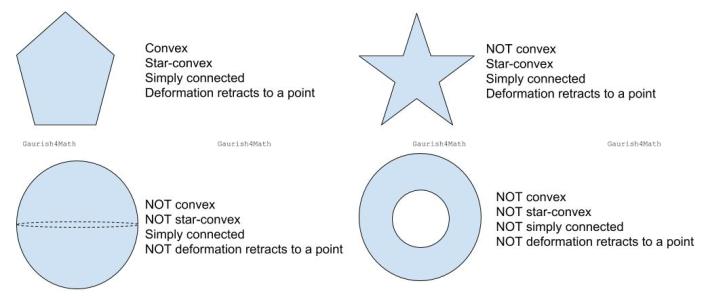
Various examples to illustrate the interdependence of these terms. Shown here are pentagon, star, sphere, and annulus.
A stronger version of Jordan Curve Theorem, known as Jordan–Schoenflies theorem, implies that the interior of a simple polygon is always a simply-connected subset of the Euclidean plane. This statement becomes false in higher dimensions.
The n-dimensional sphere is simply connected if and only if
. Every star-convex subset of
is simply connected. A torus, the (elliptic) cylinder, the Möbius strip, the projective plane and the Klein bottle are NOT simply connected.
The boundary of the n-dimensional ball , that is, the
-sphere, is not a retract of the ball. Using this we can prove the Brouwer fixed-point theorem. However,
deformation retracts to a sphere
. Hence, though the sphere shown above doesn’t deformation retract to a point, it is a deformation retraction of
.
Footnotes
- In general, a convex set is defined for vector spaces. It’s the set of elements from the vector space such that all the points on the straight line line between any two points of the set are also contained in the set. If
and
are points in the vector space, the points on the straight line between
and
are given by
for all
from 0 to 1.
- A path from a point
to a point
in a topological space
is a continuous function
from the unit interval
to
with
and
. The space
is said to be path-connected if there is a path joining any two points in
.
- There exists a continuous map
such that
restricted to
is
. Here,
and
denotes the unit circle and closed unit disk in the Euclidean plane respectively. In general, a space
is contractible if it has the homotopy-type of a point. Intuitively, two spaces
and
are homotopy equivalent if they can be transformed into one another by bending, shrinking and expanding operations.
- Then a continuous map
is a retraction if the restriction of
to
is the identity map on
.
- A homotopy between two continuous functions
and
from a topological space
to a topological space
is defined to be a continuous function
such that, if
then
and
. Deformation retraction is a special type of homotopy equivalence, i.e. a deformation retraction is a mapping which captures the idea of continuously shrinking a space into a subspace.
You must be logged in to post a comment.